Adriaan Bax, Ph.D., NIH Distinguished Investigator
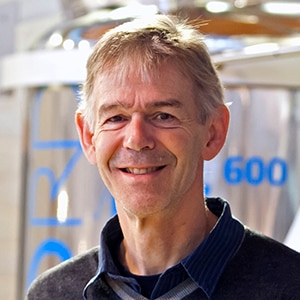
Professional Experience
- Robert A. Welch Award in Chemistry, 2018
- National Academy of Sciences (NAS) Award for Scientific Reviewing, 2018
- Professor by Special Appointment, Radboud University, Nijmegen, The Netherlands, 2014-2019
- Hinshelwood Lecturer, Oxford, England, 2012
- NIH Distinguished Investigator, Laboratory of Chemical Physics, NIDDK, NIH, 2005
- Chief, Section on Biophysical NMR, Laboratory of Chemical Physics, NIDDK, NIH, 1991
- Visiting Scientist, Laboratory of Chemical Physics, NIDDK, NIH, 1983
- Post-Doctoral Associate, National Solid State NMR Facility, Colorado State University, Fort Collins, Colorado, 1982 -1983
- Research Fellow, Department of Applied Physics, Delft University of Technology, The Netherlands; and Physical Chemistry Laboratory, Oxford University, England, 1978 - 1981
- Ph.D., Applied Physics, Delft University of Technology, 1981
- B.S., Applied Physics, Delft University of Technology, 1978
Research Goal
The primary goal is to extend the capability of currently available nuclear magnetic resonance (NMR) methods to study the structure, dynamics, folding and misfolding of proteins, as well as static and dynamic interactions between macromolecules. A secondary goal is to use these novel technologies to gain insights into the nucleating events of amyloid diseases.
Current Research
Our research has a primary focus on the development of novel, enhanced NMR techniques and approaches that will provide improved access to the structural and dynamic aspects of biomolecular systems and their interactions.
The methodology research focuses on the following five areas:
- Improving the accuracy of biomolecular structures determined by NMR data. We are especially interested in developing the following: (a) better measurement techniques for interproton distances and dihedral angles from nuclear Overhauser effects (NOE) and J couplings, and (b) further development of quantitative relations between molecular structure and chemical shifts as well as chemical shift anisotropy.
- Characterization of long-range intramolecular order by the measurement of dipolar couplings and rotational diffusion anisotropy. Such methods are aimed at addressing the main shortcoming of conventional NMR methods—the fact that they provide strictly local structural constraints. These methods will be particularly useful for studying molecular machines with partially flexible hinges, such as found in molecular motors, and allosterically controlled enzymes.
- Using novel technology that allows fast switching of hydrodynamic pressure inside an NMR sample tube by several kilobars, we can modulate the folding-unfolding equilibrium of proteins and collect real-time data on the complex process by which proteins can switch their structure. This research is of interest to both enhancing our understanding of the all-important protein folding process, but also for gaining experimental insights in misfolding, a process that underlies amyloid diseases.
- Development of NMR approaches that facilitate and expedite the structure determination process, taking advantage of the large structural databases developed by X-ray crystallography and NMR methods. Using such database approaches potentially makes it possible to study the structures of larger molecular weight systems with relatively little effort.
- Mechano-sensing and force probing by NMR methods. We are exploring the effect of externally applied force on protein NMR chemical shifts and protein structure. NMR spectroscopy holds strong potential to probe the effect of weak forces (<2 pN) at an atomic level precision, thereby providing a unique complement to other biophysical methods for measuring the effect of such forces by optical trapping.
Applying our Research
Deeper understanding of macromolecular structures and their dynamic properties will improve our knowledge of how biology works at the molecular level and provide insights into complex diseases, including a number of amyloid diseases, incl. Alzheimer’s, diabetes, and Parkinson’s disease. Work is geared in particular at obtaining an in-depth understanding of the initial nucleating events that lead to oligomer and fibril formation.
A second area of intense focus is on obtaining a better understanding of proteins involved in HIV replication. In particular, we are focused on the HIV-1 surface glycoprotein gp41 and its structural transition that occurs when the virus engages and enters a human host cell.
Need for Further Study
Further technological improvements are needed at all levels, ranging from very fundamental issues such as the relation between chemical shift and local structure, enhanced methods to extract chemical shift and other structural restraints from complex NMR spectra, to the development of computationally sophisticated procedures at the interface between molecular modeling and classical experimental structure determination. Together, this will expedite the process and extend the applicability of NMR spectroscopy to ever larger and more complex systems.
Select Publications
- Observation of β-Amyloid Peptide Oligomerization by Pressure-Jump NMR Spectroscopy.
- Barnes CA, Robertson AJ, Louis JM, Anfinrud P, Bax A.
- J Am Chem Soc (2019 Sep 4) 141:13762-13766. Abstract/Full Text
- Transient lipid-bound states of spike protein heptad repeats provide insights into SARS-CoV-2 membrane fusion.
- Chiliveri SC, Louis JM, Ghirlando R, Bax A.
- Sci Adv (2021 Oct 8) 7:eabk2226. Abstract/Full Text
Research in Plain Language
The molecules that medical researchers want to study the most are too small to be observed by light microscopes. Nuclear magnetic resonance (NMR) relies on the magnetic properties of the nucleus of certain atoms to determine physical and chemical properties of the molecules in which they are contained. Our lab is developing techniques that make the information derived from NMR more precise and that allow researchers to conduct a wider range of experiments to learn about the structure of important molecules, how they acquire the structures they have, and how alterations in their structures affect their function.